THE ECONOMICS OF WATERSHED MANAGEMENT - PROBLEMS AND RECOMMENDATIONS
FOR PROJECT ANALYSIS
Kenneth N. Brooks, Fellow, East-West Center (1983-84)
and Associate Professor
College of Forestry, University of Minnesota
and
Hans M. Gregersen, Professor
College of Forestry, University of Minnesota
St. Paul, MN 55108 USA
SUMMARY
The economic aspects of watershed management practices need to be quantified
and better understood by decision makers if we are to see such practices
become integral parts of resource development projects. Economic analysis
of watershed management projects/ programs requires a comprehensive evaluation
of many resources both at the site of implementation and at downstream
sites as well. Quantifying the benefits derived from watershed practices
requires that an array of technical relationships be developed. For example,
vegetation management and erosion control practices should be related
to upland productivity and downstream flows of water and sediment. In
addition, the benefits derived from watershed practices must be valued
in terms that are meaningful to decisionmakers. Some benefits from watershed
management practices cannot be readily valued as marketed goods. This
paper examines the problems and unique dimensions which place watershed
analyses outside of the more conventional economic approaches.
INTRODUCTION
Well developed and comprehensive watershed management programs and projects
are rarely found in the heavily populated mountains of the Asia/Pacific
region. This is largely due to the low priority given to such activities
by decision makers (FAO, 1982). A lack of awareness of potential impacts,
the low visibility of watershed projects, and the long-term nature of
such projects (Sfeir-Younis, 1983), may in part explain the low priority
given to them. Our feeling is that watershed projects have not been clearly
and convincingly shown to be economically sound, socially acceptable,
and politically attractive.
The purpose of this paper is to examine the economic aspects of watershed
management projects, illustrate and develop an understanding of the benefits
derived, examine the problems and unique dimensions of watershed analyses,
and consider ways in which such analyses can more effectively be performed
and presented to decision makers. In accordance with the theme of this
meeting, we also will point out the need for an integrated approach; decisions
are usually based on a complement of economic, social/cultural and environmental/ecological
factors. Developing projects that are economically sound is of great importance
to decision makers, but it must be recognized that economics is but one
dimension to consider in assessing alternatives.
THE PROBLEM
As a starting point for this discussion, we will initially consider the
nature of watershed management projects and focus on the problems and
unique aspects of evaluating them in economic terms. We intend to get
at the heart of the question of why watershed management activities and
projects are not generally incorporated into rural development projects.
More specifically, why are watershed projects not viewed as economically
sound parts of resource development in both upland and downstream areas?
Watershed management should be considered synonymous with multiple use
or multiple resource management, but with the condition that such use
and management be in harmony with sound soil and water conservation principles.
Watershed projects involve one or more practices (Table 1) that are often
spatially diffuse and take place over a long period of time. In the simplest
case, watershed projects may entail a very specific set of well understood
actions - - such as in the case of watershed rehabilitation. Rehabilitation
may be undertaken to protect downstream, high investment projects such
as reservoirs. Structures may be built to stabilize channels or to halt
gully erosion. Reforestation and other vegetative measures, including
agroforestry practices, should be integral parts of the project. However,
without long-term land management commitments, the effective life of rehabilitation
practices is generally short lived.
Table 1. Examples of practices that may be evaluated within watershed
rehabilitation or management projects (modified from Gil, 1979).
Rehabilitation of Uplands |
|
Agricultural Lands |
Agroforestry practices a/
Contour cultivation
Terracing |
Wildlands |
Gully control structures
Revegetation - reforestation
Slope stabilization
Sand dune fixation
Wetland drainage |
Management of Uplands |
|
Agricultural Lands |
Water spreading
Agroforestry practices
Shelterbelts
Brush control
Fencing
Strip cropping |
Wildlands |
Grazing management - fencing, etc.
Protection of critical areas buffer strips, etc.
Forest protection fire control
Zoning for hazards landslides, floods, etc.
Road - skid trail guidelines & control
Regulated forest harvesting |
Associated Water Resource Development. |
Wells and spring development
Water harvesting
Mini-hydro projects
Reservoirs
Infiltration galleries
Diversion systems
Channel improvement
Aquaculture development |
a/ Many practices such as agroforestry and cultivation techniques
may be considered as either rehabilitation or management.
Some of the most important aspects of watershed management projects are
often overlooked because they are less visible than structural measures
such as dams and gully plugs. An array of non-structural practices are
needed which complement structural measures but which promote sustained
productivity as well (Table 1). The importance of non-structural practices
in relation to watershed management benefits often is difficult to convey.
If the connection is not made, then the result can be low priority given
to these project elements.
Watershed projects affect upstream and downstream areas and usually affect
more than one community, governmental body, institution and administrative
level. There is rarely a single administrative unit that is solely responsible
for watershed management in a given project area. Usually, resource management
and development programs for forestry, water resources and agriculture
are the responsibility of several independent agencies or institutions.
In some cases, one group bears the costs and another reaps the benefits.
For example, upland rehabilitation and reforestation may be the responsibility
of-landowners and a forestry agency, but major beneficiaries of erosion
control may be an energy (hydropower) agency and water resource (irrigation
water) users downstream. The separation of those who pay from those who
benefit results in difficult administrative problems and usually requires
considerable cooperation, coordination, and in some cases compensation
among institutions.
The above points indicate, in a general way, some of the reasons why
watershed projects are not viewed as high priority projects in the eyes
of decision makers. Several other pertinent considerations include:
i) Watershed projects require long-term commitments of resources in regions
where resources are scarce; decision makers tend to favor projects that
show more immediate and visible benefits.
ii) Watershed management and rehabilitation often occurs in remote areas,
away from political and economic or financial centers, and do not, therefore,
attract widespread support.
iii) Watershed projects may be geared toward preventing potential losses
rather than increasing benefits. While losses prevented are also benefits,
they are less attractive to decision-makers interested in more immediate
stimulation of local or national economies.
iv) The economic and financial benefits and feasibility of watershed
projects and management programs have not been effectively demonstrated.
The last item will be examined more closely. Certainly excuses that can
be offered include lack of knowledge or inability to identify and quantify
all pertinent inputs and outputs associated with projects. Likewise, the
difficulty of assigning values to many of the benefits and the need for
both economic and financial considerations place such analyses outside
of the more conventional approaches and are problems that will be discussed
further . To help understand the benefits of watershed management, a framework
for analyzing the economics of watershed projects will be briefly reviewed.
FRAMEWORK FOR ECONOMIC ANALYSIS
Any analysis of watershed management projects must objectively examine
and compare inputs needed and goods and services produced "with and
without" the project/program. Using Dixon's (1984) framework (Figure
1) as a point of reference, all goods and services that are affected by
the project should first be identified and then input-output relation
ships quantified. The steps needed for such an analysis, discussed by
Gregersen and Contreras (1979), have been applied to watershed management
projects by Gregersen and Brooks (1980) and Brooks et al. (1982). Briefly
these steps are:
Figure 1. Relationship between the goods and services associated with
watershed management projects and location (adapted from Dixon, 1984,
and Hamilton and Snedaker, 1984).
|
LOCATION OF GOODS AND SERVICES
|
|
UPLAND WATERSHED |
DOWNSTREAM AREAS |
TYPES OF GOODS
AND SERVICES |
MARKET |
I |
II |
NON-MARKET |
III |
IV |
Quadrant I - Food crops, forage for livestock, animal products, fuelwood,
pulpwood, lumber, other wood products, minerals, water, fisheries;
Quadrant II - Water for drinking, fisheries, irrigation, hydroelectric
power generation, navigation, recreation, municipal & industrial supplies;
flood control benefits; sediment control for avoiding losses of reservoir
benefits, etc.
Quadrant III - Aesthetic values; wildlife habitat protection; health
benefits of high quality water supplies; protection of aquatic ecosystems;
landslide mudslide control (minimization); preservation of gene pools
(natural vegetation);
Quadrant IV - Protection of downstream riparian and aquatic ecosystems;
high quality water for recreation-aesthetic values.
i) Develop technical relationships and physical flow tables for the conditions
"with and without" the project;
ii) Estimate the values and flow of values for the inputs and outputs
determined from step 1;
iii) Calculate the measures of project worth, such as the benefit-cost
ratio, economic rate of return and net present worth; and
iv) Test the sensitivity of the measures of project worth to changes
in the assumptions.
We do not intend to delve into economic theory or reasons for the above
steps. The reader is directed to the above references and to Gottinger
(1983) and Hufschmidt et al. (1983) for a more complete discussion of
approaches to economic analysis of resource development projects and programs.
Our purpose is only to point out where the greatest deficiencies and bottlenecks
exist in performing economic analyses of watershed management projects,
to describe the unique aspects of watershed project evaluation, and to
suggest ways of improving project analysis and evaluation.
QUANTIFYING TECHNICAL RELATIONSHIPS
Much has been written about the economic, social and environmental losses
caused by watershed degradation. Deforestation, fire, overgrazing, and
shifting cultivation are usually blamed for degradation and resulting
downstream impacts such as flooding and reservoir sedimentation. Many
of the reported impacts are real, but some impacts attributed to upland
degradation have been exaggerated. Part of the problem before us is to
identify and quantify real impacts and their causes. Technical relationships
must be developed to relate system inputs and outputs for conditions "with
and without" the project.
The With and Without Approach
The existing situation or "without project" condition must
first be defined and evaluated for the watershed using available inventory,
monitoring and survey data. Even in developed countries, the absence of
data often constrains our analyses. Existing rates of erosion, levels
of sedimentation, water yield, water quality and even crop, livestock
and wood production can be difficult to quantify -- particularly under
subsistence farming. At this point, the value of an experienced team of
resource specialists cannot be overemphasized. Opinions and judgments
of competent professionals are needed to fill in the voids created by
the absence of data. Likewise, the judgment and experience of such professionals
are needed to estimate the long-term implications on resource outputs
if the watershed condition is permitted to continue to degrade, i.e.,
without the project.
The next step in the analysis requires that "with project"
conditions be defined; the effectiveness of rehabilitation and management
practices must be expressed in quantitative terms. But there is frequently
a lack of quantitative relationships between forest alterations or land
use changes and hydrologic or soil responses, particularly in the tropics
(Hamilton with King, 1983). Even where functional models of watershed
systems exist, the scarcity of data for model calibration and verification
represents a serious constraint.
The types of technical relationships needed for watershed analyses vary,
but in every instance the watershed condition or action should be related
to physical outputs that have meaning in economic, social or environmental
terms. Examples of technical relationships needed for "with and without"
project conditions are listed in Table 2. An example of one form of such
relationships is shown in Figure 2. In this illustration, the level of
land use or perturbations (X) can have both positive and negative effects
on some measure of productivity (Y). By developing such a production function
approach to biophysical relationships, as suggested by Smathers et al.,
(1983), the relationships are easily understood and used in economic analysis.
A hypothetical example might relate the level of nutrient export (X) in
Kg/year associated with upland disturbance to fisheries production (Y)
in Kg/year, of a downstream reservoir. The initial status of the reservoir
determines the effects of increased levels of nutrient loading. If the
reservoir is nutrient-poor (point XA ), there may be an increase
in fisheries production caused by increased nutrient loading (moving from
point A to point B). Eventually, additional nutrient input (greater than
XB) will reduce fisheries production. If nutrient loading is
allowed to proceed, a point may be reached (XC) where irreversible
effects occur; the reservior is so eutrophic that life can no longer exist
and the system cannot recover without some major rehabilitation effort.
In this example, the project effects on nutrient export would need to
be known, along with the present status of the reservoir.
With all the research that has taken place over the years, one may ask
why functional relationships like Figure 2 are not better known for the
important watershed outputs and attributes. One problem is that many researchers
have not been aware of, or sensitive to, the types of relationships and
information needed by economists and decision makers. Research has often
been isolated from the management and decision making environment. Multidisciplinary
research must be coordinated and targeted for practical results usable
in making decisions.
Although the precise shape and magnitude of production functions may
not be known for variables such as those listed in Table 2, approximations
can usually be developed from experience and from work done elsewhere.
For our discussion, the kinds of technical relationships for upland watersheds
will be considered separately from downstream areas. Such a separation
serves another purpose as well; it helps identify possible inequities
that arise from watershed projects because of the spatial distribution
of costs and benefits. The distribution of costs and benefits must be
dealt with in the final analysis even though the overall project may be
economically feasible.
1 See paper by Hamilton in these proceedings.
Table 2. Examples of technical relationships needed to perform economic
analysis of watershed management projects; under with and without project
conditions.
Dependent Variables
Y |
Independent Variables
X |
Uplands
(1) Annual erosion rates (tonnes/ha) |
Land use/watershed practices; e.g., as
characterized by Universal Soil Loss Equation |
(2) Crop production (Kg/ha)
Wool production (Kg/ha)
Meat production (Kg/ha)
Wood production (m³) /ha) |
Annual erosion rates (tonnes/ha) for each
land-use/watershed practice |
(3) Annual water yield (m³/ha)
Ave. minimum 5-day flow (m³/sec)
Ave. annual peak discharge (m³/sec)
Frequency of landslides* |
Change in forest cover, as % of watershed |
(4) Wildlife habitat diversity or numbers
of species present |
% watershed forested, rangeland and cultivated |
Downstream
(5) Sedimentation rates at reservoir or channel (m³/yr) |
Annual erosion rates (tonnes/ha) from above |
(6) Annual loss of hydropower generation
capacity, loss of irrigation capacity, etc. |
Sedimentation rates (m³/yr) |
(7) Frequency of flooding* |
Stormflow-stream stage relationships under
different channel conditions |
(8) Fisheries production in reservoirs/lakes
(Kg/yr) |
Nutrient loading from upland watersheds
(Kg/yr) |
(9) Average annual losses ($) due to flooding |
Frequency of flooding* and sedimentation
of channels |
* Technical relationships for determining land use impacts on landslides
and flood frequency and associated damages require involved and complex
analyses. Methods of performing hazard analysis for such events are presented
by Petak and Atkisson (1982).
Figure 2.
Example of a generalized technical relationship for watershed systems
that is in the form of a production function as suggested by Smathers
et al. (1983). "A" represents no land disturbance or zero perturbations,
"B" is the maximum productivity of Y at some specified level
of use or perturbation (XC) and "C" is where the
level of use or perturbations (XC) results in irreversible
impacts on productivity.
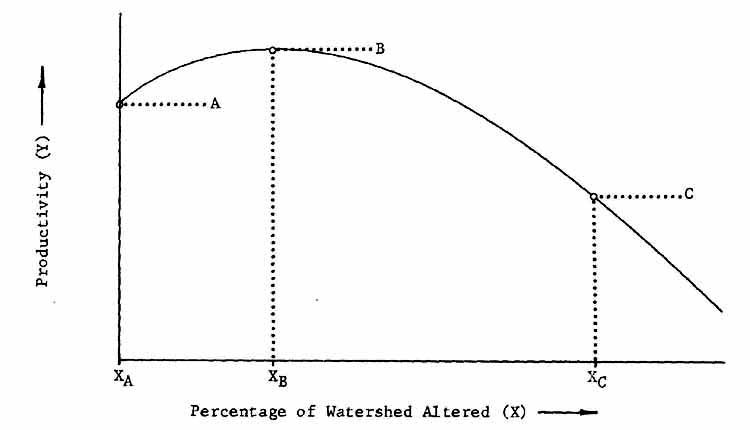
Upstream-Upland Relationships
Upland watersheds can be managed to provide multiple goods and services
for local and downstream communities. People typically subsist in the
uplands of developing countries, deriving much of their food, fuel and
animal product needs from them.
In the past, losses in productivity due to land use were taken care of
by traditional land use patterns such as shifting cultivation and nomadic
grazing. Indigenous farmers and herdsmen understood the signs of reduced
productivity and simply moved to new, more productive sites. Today, population
pressures in many developing countries cause excessive and repeated use
of watersheds without an adequate fallow period (as discussed earlier
by several authors). Shortened burning and cultivation cycles in tropical
forested watersheds are depleting the nutrient capital of many uplands
to the extent that active rehabilitation measures are needed.
Upland Productivity
Production functions for variables listed in Table 2 could be theoretically
developed to relate upland productivity, e.g., Kg/ha of food, cubic meters/ha
of fuelwood, and Kg/ha of wool, to the percentage of the watershed cultivated
or grazed. Again referring to Figure 2, if we started with a totally undisturbed
watershed (point A), increased levels of land use could result in increased
productivity (up to point B), at which time the level of land use begins
to exceed the capacity of the watershed. Further use results in soil erosion
and loss of productivity until, if use is not diminished, a point of irreversibility
(XC) is reached. The extreme situation would be that all soil
is lost from the site. By adding fertilizers and implementing soil conservation
practices on the watershed, productivity may eventually shift to the left.
This new state may change the shape and magnitude of the relationship
altogether. This is an example of where the soil specialist and resource
economist need to work together to develop the appropriate technical relationship.
The loss of topsoil from farmlands can usually be related to either loss
of crop productivity or costs of fertilizers needed to sustain productivity.
Wildland watersheds present a more difficult problem. The levels of productivity,
particularly for subsistence farming, may be difficult to determine. Also,
because erosion in uplands does not occur uniformly the loss of soil nutrients
may be difficult to assess. Gully erosion and mass soil movements leave
portions of a watershed with essentially "zero" productivity
for some time period.
Soil loss can have serious hydrologic consequences in addition to the
loss of vegetative productivity. The amount of soil water available for
plant growth on the site is reduced which may be more serious than loss
of nutrients. The resulting increase in runoff to downstream areas can
result in many other losses as well.
An example of the type of information needed to evaluate the economic
impacts of soil erosion on productivity is given by Tejwani and Babu (1982).
in an agricultural setting near Dehra Dun, India, the effects of topsoil
removal on the yield of rain-fed maize were evaluated. For every one cm
of topsoil removed, maize production dropped 100 Kg/ha the first year,
52 Kg/ha the second year, and 51 Kg/ha the third year. The value of protecting
topsoil from erosion from an upland perspective can be readily determined
from such data. However, the total value of protecting topsoil must include
downstream benefits as discussed later.
Non-market Considerations
Several other types of benefits can be attributed to watershed management
projects or programs in upland areas that are not related so closely to
marketed products. For example, the amount and quality of drinking water
can be enhanced for upland inhabitants. Benefits could include better
health of people and livestock, which may indirectly be related to productivity,
as well as improved fisheries production. The aesthetics of streams and
rivers in the area may be enhanced and aquatic habitat maintained or improved
as well. Such nonmarket, environmental benefits should be considered in
an economic analysis, but are more difficult to quantify and value as
pointed out by Gottinger (1983) and Hufschmidt et al. (1983).
The problem of irreversible consequences, in some cases a result of doing
nothing, should be evaluated carefully by decision makers. An example
of irreversibility, in the truest sense of the word, would be the destruction
of habitat for an endangered species resulting in that species becoming
extinct. identifying such possibilities in most developing countries is
difficult, as is determining the cost of such impacts. In the instance
of excessive soil erosion from upland watersheds, soil loss may not be
absolutely irreversible, but in reality, hundreds of years may be required
for the system to recover unless active rehabilitation measures are implemented.
The level of degradation at which the ability to rehabilitate a watershed
becomes physically and economically unrealistic is sometimes hard to define.
Nonmarket considerations for upland watersheds may include relationships
among management actions and the occurrence of events such as landslides,
mudflows, soil creep and flash floods. We know that mountainous regions
are susceptible to such events no matter what the types of land use. We
also know that, to some extent, the frequency of occurrence and/or the
magnitude of damages caused by such events can be aggrevated by improper
land use practices, improper location of roads, poor drainage systems,
etc. The benefits of avoiding or minimizing losses can sometimes be related
to the monetary value of such things as buildings, farm equipment, or
farmland. However, risks to human life present another dimension which
is beyond the scope of our discussion. The reader is referred to Gottinger
(1983) for a detailed discussion of analyzing human life, human capital
and hazard assessment.
Although catastrophic events such as landslides cannot be altogether
prevented, management practices can be implemented that help reduce annual
damages and losses and at the same time provide needed products. For example,
planting deep-rooted trees and/or shrubs on steep slopes, as parts of
fuelwood or agroforestry projects, can provide multiple benefits such
as slope stabilization, fodder, fuelwood, and other wood products. Quantifying
benefits in terms of losses avoided requires a site specific analysis
on the watershed -and even then considerable uncertainty exists.
The preservation of natural ecosystems, scenic areas, and wildlife habitat
can be important attributes of watershed projects. In some cases, preservation
may be justified on the basis of multiple benefits, some economic and
some not. For example, preserving natural forests on excessively steep
slopes can also have value in stabilizing slopes and minimizing sediment
delivery. Likewise, protecting riparian vegetation can protect valuable
wildlife habitat while stabilizing stream banks and reducing sediment
delivery to downstream areas. We cannot ignore, however, the fact that
preservation of some ecosystems is in the best interest of society as
a whole, and may not readily be evaluated solely on the basis of economics.
Downstream Relationships
Many watershed projects are implemented primarily to protect major downstream
facilities such as reservoirs and irrigation systems. A combination of
activities, as indicated in Table 2, may be initiated before, during and
after the construction of large, high investment projects. Their primary
function may be to reduce sedimentation at downstream reservoirs or channels.
Erosion and Sedimentation
The relationship between upland erosion (and erosion control practices)
and downstream sedimentation is usually poorly understood. The reasons
are many and include:
i) Data on rates of erosion are not available.
ii) Downstream sedimentation results not only from upland surface erosion,
but also from channel erosion and mass movements (such as landslides)
into the channel.
iii) Channel characteristics and distances between affected watersheds
and downstream reservoirs determine the quantities and timing of sediment
delivery (thus, adding complex time and space dimensions to the analysis).
iv) Sedimentation caused by natural, catastrophic floods and landslides
can be excessive, but are probabilistic in nature and difficult to quantify
under "with and without" project conditions.
Although precise models of upland erosion and downstream sedimentation
are rare, several general methods are available, such as linking the Universal
Soil Loss Equation (USLE) with delivery ratio or transport models. If
some data on sedimentation rates (from pond or reservoir surveys) are
available, rough approximations can be developed, as described in the
following example.
The Loukkos example illustrates one of the most commonly cited justifications
for developing major erosion control and watershed projects, that of protecting
large multipurpose reservoirs. Even so, major water resource projects
continue to be planned and constructed without full appreciation of the
importance of upland watershed management to their economic feasibility.
Gregersen and McGaughey (1983) estimated that over $US 800 million will
be needed for non-structural watershed management activities in Latin
America over the next 20 years just to protect anticipated new hydroelectric
reservoir projects.
Example. Erosion control practices were implemented in the Loukkos Basin,
northern Morocco, to extend the life of a large reservoir that was designed
to supply irrigation water, hydroelectric power, municipal and industrial
water supplies and provide flood control benefits (Brooks et al. 1982).
Sedimentation rates for "without" project activities were estimated
from sediment surveys in nearby reservoirs and ponds. These rates were
then related to upland erosion rates by applying the USLE and a reasonable
delivery ratio (.39). The existing upland erosion rates were considered
to represent the "without" project condition. Values in the
USLE were then modified to estimate changes in erosion rates caused by
erosion control practices. Using the same delivery ratio, changes in downstream
sedimentation at the reservoir site were quantified. It was assumed that
50 percent of reservoir sedimentation originated from upland erosion,
the other 50 percent derived from the channel itself. Benefits and costs
in both the upland watershed and the reservoir site were then determined
for "with and without" project conditions. Benefits included
upland increases in productivity of agricultural, livestock, and wood
products, and downstream benefits of irrigated crop losses avoided (extending
the reservoir life). Because these benefits far exceeded the costs, other
benefits attributed to the project, e-g., hydropower losses avoided, and
the sustaining of flood control capabilities -- components that are more
difficult to quantity - were not evaluated.
To compensate for uncertainty in some of the assumptions, a sensitivity
analysis was performed. Even with major shifts -- up to 25 percent higher
costs and 25 percent lower benefits -- the project represented an economically
efficient use of resources. If this simplified analysis had shown the
project to be only marginally feasible, then a more detailed and complete
assessment would have been made. This example illustrated that a simple
economic analysis can be made of watershed projects in which data are
scarce.
Flooding
Flooding represents a major economic loss to downstream communities and
is said to increase as a result of upland deforestation and watershed
degradation. The extent to which watershed condition affects flooding
is somewhat controversial. Lack of a clear definition of terms and of
cause and effect relationships has lead to much confusion. As pointed
out by Hewlett (1982), forest cutting activities in the United States
have not lead to increased flood flows in major streams. Most research
has shown that forest clearcutting followed by regeneration has a short-lived
effect on stormflow volumes and peaks, and this effect becomes less evident
as the magnitude of the precipitation event increases. Further, the percentage
of major watersheds that are clearcut at any point in time is usually
small. In brief, commercial clearcutting may increase peak discharge and
volumes from small watersheds for storms with moderate precipitation amounts,
but has little effect on major flood events, i.e., those associated with
a 50- to 100-year recurrence interval or greater.
Widespread and more permanent conversion from forest cover to croplands
or pastures, particularly if accompanied by severe erosion, may have more
serious implications than suggested by Hewlett (1982). For example, widespread
conversion from forest cover to cultivated lands can result in: i) increased
surface runoff and higher peak flows, ii) higher volumes of runoff, and
iii) sedimentation of channels and, consequently, a reduction of channel
capacity to convey runoff. If all three occur, the elevation (or stage)
of streamflow events in channels will tend to be higher under conditions
of denuded watersheds than forested watersheds. As channels fill with
sediment, the frequency with which streamflow exceeds its banks will increase.
Whether floods result in serious damages depends largely on development
and human occupancy on the flood plain.
The question of interest for the economic analysis of flood flows is:
are there truly flood control benefits that can be attributed to watershed
management projects, particularly those involving re-afforestation? Being
objective, we have to answer with a definite "sometimes" and
"to a limited extent." As pointed out above, watershed condition
can influence the magnitude of peak flows and corresponding streamflow
stages associated with moderate sized storms. Large and devastating floods
along major river systems, however, are principally caused by excessive
rainfall and/or snowmelt; they will occur no matter what the watershed
condition. Therefore, the development of technical relationships to evaluate
flood damages with and without watershed management projects, requires
a detailed hydrologic study of upland watersheds and their linkage to
downstream flood plains. Changes in the flood frequency curve due to project
activities must first be determined and then these changes equated to
changes in annual flood damages.
Water Quality
Maintenance of high quality water to downstream communities is another
benefit attributed to proper watershed management practices. High quality
water is usually associated with forested watersheds that are well managed,
have sparse human populations, few grazing animals, and minimal soil erosion.
Extensive use of watersheds can lead to polluted drinking water supplies,
reduced production of fisheries, reduced water-based recreational opportunities
and adverse effects on aquatic ecosystems. Relating upland management
activities to downstream water quality has similar, but even more difficult
problems than previously discussed for erosion-sedimentation relationships.
DETERMINING VALUES FOR BENEFITS AND COSTS
Placing monetary values on watershed project inputs and outputs follows
the step of quantifying technical input-output relationships discussed
in the previous section. Methods for determining the value of watershed
inputs and outputs are discussed by Gregersen and Contreras (1979), Brooks
et al. (1982), Hufschmidt et al. (1983), and references cited therein.
Here, we only intend to point out some of the important considerations
and difficulties in this process.
Some watershed attributes and project benefits simply cannot be assigned
acceptable monetary values. When such elements are identified, they should
be discussed in qualitative terms such as social/cultural benefits or
environmental/ecological benefits in project documentation. This does
not imply that such benefits are not of value. All benefits should be
considered in the decision-making process, but not always on the basis
of conventional economic analysis.
Many of the outputs from upland watersheds (quadrant I in Figure 1) can
readily be assigned monetary values (although for subsistence farming
the process may not be straightforward). Outputs could include crops,
forage, meat, wool, fuelwood and other wood products. Watershed projects
should increase the monetary benefits of these outputs because incentives
are then created for upland inhabitants, i.e., those who have to carry
out the upland practices. Even though the main thrust of a project may
be to protect downstream reservoirs, the success of the project in the
long run may hinge on financial benefits acting as incentives for upland
inhabitants.
Determining the value of goods and services in quadrants II, III and
IV or Figure 1 is more difficult for the analyst than those in quadrant
1. In some cases, categorizing benefits is arbitrary; some benefits may
reasonably be included in more than one quadrant and therefore, represent
different types of values and may occur at different locations. For example,
water quality benefits may be realized in both upland and downstream areas
and can also be considered as market and non-market benefits. Drinking
water has monetary benefit for both upland and downstream areas. Millions
of dollars may be spent by governments and municipalities to remove impurities
from water so that it may be used for drinking and other municipal and
industrial purposes. The benefits of high quality water may be determined
and valued in terms of treatment costs avoided, or in terms of the costs
of developing alternative water supplies. On the other hand, improved
drinking water may have value in terms of improved health (reduced health
care costs) and welfare of people. Monetary values may be derived on the
basis of lower health care costs, greater number of work hours or greater
worker output. High quality water likewise may have non-market value in
terms of maintenance or improvement of aquatic ecosystems and streamside
(riparian) ecosystems in upland and downstream areas.
Some of the problems of "valuing" benefits of environmental
projects presented by Gottinger (1983) and Hufschmidt, et al. (1983),
but with special emphasis on watershed projects, are summarized below:
i) Irreversible consequences - societies may be willing to pay a premium
that exceeds the expected value of such a loss to prevent that loss. From
a general welfare perspective, Gottinger suggested that irreversible losses
be considered losses of "future options available." Such losses
may be deemed important enough to serve as a constraint to the project;
for example, project objectives may explicitly state that an endangered
species will be protected. The problem does not then have to be dealt
with in the economic analysis, but certainly constrains the alternatives
that are available. The "value" might then be examined in light
of the project cost "with and without" the constraint imposed.
ii) Collective or public goods and services - non-market attributes of
projects such as aesthetics or public health previously discussed.
iii) The preservation of ecosystems - environmental and multiple-use
benefits associated with many natural ecosystems that are not readily
evaluated with market approaches. Hufschmidt et al. (1983) devote one
chapter to valuing such environmental benefits in monetary terms.
iv) Soil loss over time - the value of incremental soil losses over long
periods of time represents a rather unique aspect of watershed project
evaluation. Soil erosion on an upland watershed may be difficult to quantify
and value. Even if quantified, the annual value of soil lost would likely
be small. The accrued value over many years plus the "irreversible"
nature of long term erosion suggest that the true value to society may
not be considered with normal "farmland" analysis.
v) Human life - this represents a major consideration in many watershed
projects, particularly in terms of the effects of better drinking water,
sanitation considerations, and protection against the hazards of floods
and landslides. Gottinger (1983) provides useful suggestions for approaching
this sensitive subject.
EVALUATING THE PROJECT
The last two steps in the economic analysis are to calculate the measures
of project worth and then test the sensitivity of such measures to changes
in the assumptions used (i.e., testing for effects of uncertainty). Several
important points concerning watershed projects need to be considered here.
A number of assumptions have been made up to this point in the analysis;
uncertainty exists for many of the technical relationships and values
calculated. A project planner can consider uncertainty by examining the
consequences of using shifts in the relative values of benefits and costs
and using different discount rates. For example, fuelwood prices may be
relatively high compared to petroleum prices (or other alternatives) under
present conditions, but what happens if petroleum prices double in the
next ten years? A sensitivity analysis can be useful to examine different
scenarios and anticipate changes that might occur in the future. By explicitly
stating all assumptions throughout the analysis, those which appear weakest
can be tested in such a manner. Further, sensitivity analysis can be used
to calculate breakeven or switching values, i.e., those values of inputs
and/or outputs which produce a zero net present worth.
Attributes of watershed management projects and programs that cannot
be assigned monetary values should be clearly identified and separated
from economic factors in the final evaluation. Social/cultural values
and environmental/ecological values should be considered as separate entities.
The evaluation of project alternatives is then based on all aspects and
values of the project. As pointed out by Dixon in Hamilton and Snedaker
(1984), the economic analysis does not give the answer, it provides information
that should aid the decision maker in considering options available and
should be treated as but one piece of information to be used in arriving
at a decision.
CONCLUDING REMARKS
Watershed management programs and rehabilitation projects can be developed
to provide monetary benefits as well as positive environmental/ecological
and social/cultural impacts. Upland development, if carried out using
sound watershed management principles, can offer an array of economic
benefits to both upland watershed inhabitants and downstream communities.
Sustained resource management may be achieved, benefiting upland rural
poor and protecting high investment projects and livelihoods in downstream
communities as well. Because of the multiple use nature of watershed management,
the benefits and costs are many and varied and are usually widely dispersed
spatially and temporally. Watershed projects require long-term commitments
of resources and often take many years to generate significant, easily
noticeable benefits. This makes economic analysis difficult.
The development of comprehensive and wide-ranging watershed management
projects/ programs in developing countries has been severely hampered
by a general lack of interest and awareness by decision-makers. In most
instances, this lack of interest may be traced to the inability to convincingly
demonstrate the economic, environmental and social values of such projects/programs.
To some extent this inability may be attributed to sparse data and limited
technical expertise and methods that are needed for comprehensive economic
evaluation. A multidisciplinary team of experienced professionals can
partially overcome data constraints by taking a systematic and integrated
approach. Such an approach involves: clearly defining the economic input-output
relationships, social benefits and environmental attributes under "with
and without" watershed project conditions; calculating measures of
project worth that are relevant to decision-makers; developing estimates
of the impacts and nature of uncertainty and intangible benefits and costs;
and presenting a clear and straightforward account of the watershed management
costs, benefits and broader implications in a development context. With
such information in hand, decision-makers should be better able to weigh
the value of watershed management practices as integral parts of all rural
development projects.
REFERENCES
Brooks, K. N., H. M. Gregersen, E. R. Berglund and M. Tayaa. 1982. Economic
evaluation of watershed projects - An overview methodology and application.
Water Resources Bulletin 18(2):245-250.
Dixon, J. A. 1984. Economic valuation: its role and use in assessment
and planning. Presented at International Training Course on Land Use Planning
and Assessment. Hong Kong, January. 13 pp.
FAO, 1982. Watershed management in Asia and the Pacific: problems, needs,
status of programmes and strategy to foster upland conservation in the
region. FAO: DP/RAS/81/053 Technical Report, Food and Agricultural Organization
of the United Nations, Rome. 66 pp.
Gil, N. 1979. Watershed development with special reference to soil and
water conservation. FAO Soils Bulletin 44. Rome. 257 pp.
Gottinger, H. W. 1983. Benefit cost analysis for environmental decision
making Angewandte Systemanalyse (Applied Systems Analysis) Bank 4/Heft
3:112-124.
Gregersen, H. M. and K. N. Brooks. 1980. Economic analysis of watershed
projects: special problems and examples. In: Economic analysis of forestry
projects; readings. FAO Forestry Paper 17 Suppl. 2, Food and Agricultural
Organization of the United Nations, Rome. pp. 133176.
Gregersen, H. M. and A. H. Contreras. 1979. Economic analysis of forestry
projects. FAO Forestry Paper 17. Food and Agricultural Organization of
the United Nations, Rome. 193 pp.
Gregersen, H. M. and McGaughey. 1983. Forest based development in Latin
America. InterAmerican Development Bank, Washington, D.C.
Hamilton, L. S. with P. N. King. 1983. Tropical forested watersheds -
hydrologic and soils response to major uses or conversions. Westview Press,
Boulder, Colorado. 168 pp.
Hamilton, L. S. and S. C. Snedaker (eds.). 1984. Economic considerations
in mangrove management. Section IV in: Handbook for Mangrove Area Management.
Environment and Policy Institute, East-West Center and the International
Union for the Conservation of Nature and Natural Resources. 123 pp.
Hewlett, J. D. 1982. Forest and floods in the light of recent investigation.
in: Proceedings of the Canadian Hydrology Symposium '82, Hydrologic Processes
of Forested Areas, Fredericton, N.B., Canada. pp. 543-559.
Hufschmidt, M. M., D. E. James, A. D. Meister, B. T. Bower and J. A.
Dixon. 1983. Environment, natural systems, and development -- an economic
valuation guide. The John Hopkins University Press, Baltimore. 338 pp.
Petak, W. J. and A. A. Atkisson. 1982. Natural hazard risk assessment
and public policy - anticipating the unexpected. Springer-Verlag, New
York. 489 pp.
Sfeir-Younis, A. 1983. Economic aspects of soil conservation programs
in less-developed countries (LDCs). Water International 8:82-89.
Smathers, W. M., Jr., C. F. Jordan, E. G. Farnworth, and T. H. Tidrick.
1983. An economic production-function approach to ecosystem management.
BioScience 33(10):642-646.
Tejwani, K. G. and R. Babu. 1982. Economic evaluation of the environmental
benefits of soil and water conservation programmes. Indian Journal of
Soil Conservation 10 (No. 2-3):80-90.

|