Blog-Water Productivity Tools and Analytics
Remote Sensing Determination of Evapotranspiration in Agriculture: Highlights and Reflections
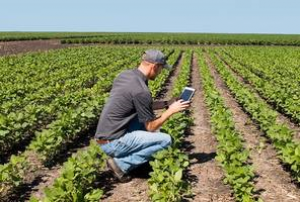
Quantitative estimates of evapotranspiration (ET) over space and time reveal the consumption of water at system scales. When the system
under investigation is in the agricultural sector, such knowledge is particularly relevant because agriculture, in general, and irrigated agriculture, in particular, are by far the most significant consumers of water. In a region facing significant
water scarcity, knowledge of ET is a vital input into sustainable water resources management, and in assessing the appropriate allocation to irrigated agriculture.
The challenge is to find feasible, accurate, timely, and affordable ways to determine ET at different operational scales, from farm fields to irrigation schemes, to groundwater systems, up to river basins, and to national levels.
There are in-field methods to determine ET that include: aerodynamic and energy balance methods (also called micro-meteorological methods, such as the eddy covariance and the Bowen ratio); use of electromagnetic scattering (such as through scintillometry); and various water balance methods (such as the lysimeters and the soil-water storage depletion methods with vertical soil-water flow measurements). These in-field methods, however, detect the ET of a limited surface area (e.g., from a few square meters to a few hectares), and therefore are mostly confined to exploratory applications typically within research and academic environments; they generally require very qualified and specialized personnel and are often highly demanding in operation, maintenance and calibration. Furthermore, scaling in-field methods up to larger areas implies the deployment of a very large number of equipment installations, which is, in the present era, prohibitive due to the instruments, labor, and maintenance costs.
In practical terms, in-field methods offer “point estimates” of ET that cannot reliably be projected across widely varying conditions.
Deriving useful spatial and temporal projections from these in-field ET determinations, from research and academic experiments, requires some types of simplified modeling approach to derive basic parameters of crop consumption, like crop coefficients (Kc). With the help of relatively simple agro-meteorological measurements in space and time (e.g., sunshine duration, air temperature, and humidity, wind speed), the reference-crop water consumption (ETo) is calculated, so that specific crop water consumptions (ETc) over a broad range of farms can be obtained as the product of ETo and Kc. Local adjustments, as well as some water-stress factors, may need to be adopted to improve the accuracy of the ETc determination.
The above crop coefficient approach, used to operate irrigation systems, also reveals its weaknesses when attempting to cover large areas due to the lack of precise knowledge of crop types and physiology of individual fields and to the lack of a sufficient number of agro-meteorological stations properly located to capture the climatic spatial variability.
Several other operations demand knowledge of ET, including: cropping systems’ water productivity; annual water accounting at national levels; assessment of agricultural drought indices; planning of water allocation to various cropping systems within the entire agricultural sector; monitoring groundwater depletion; and compliance with water rights and water transfer agreements. Most, if not all, of these uses, require knowledge of actual ET amounts rather than the potential ET amounts that are produced from the crop coefficient approach.
Essentially, we need practical and affordable methods, that allow us to monitor ET over large operational scales (from less than a few hundred to several thousand kilometers), reflecting the spatial variability of the complex land-plant-atmosphere interactions governing the ET process (from weather, topography, wind patterns, soil moisture, vegetation type, and crop husbandry), and with acceptable degrees of accuracy.
For large area coverage, that includes spatial details down to the farm-field scale, the only feasible and affordable methods for relatively accurate ET determination are through Satellite Remote Sensing (SRS).
In fact, with the advent and evolution of satellite remote sensing systems over the past two decades, along with the development of sophisticated algorithms, the determination of the spatial distribution of ET is nowadays possible and publically accessible on the web for several applications. Remaining questions are in regard to the balancing of accuracy with timeliness and affordability and in maintaining consistency of estimates over large land areas.
Depending on the specific band of the electromagnetic spectrum measured by sensors on the satellites, we have various spatial and temporal resolutions that can serve the various operational scales of ET. For the infrared or thermal band, providing the Land Surface Temperature (LST-most relevant for ET determinations), we can go from 2-5km every 15 min from the geostationary satellites (e.g., METEOSAT), down to a 375 m snapshot every day from VIIRS (Visible Infrared Imaging Radiometer Suite), to a 250 m sharpened snapshot, from a 1000 m thermal band, every day from MODIS (Moderate Resolution Imaging Spectroradiometer), to a 70 m snapshot every 1 to 5 days from ECOSTRESS (ECOsystem Spaceborne Thermal Radiometer Experiment on Space Station), to a re-sampled and sharpened 30 m snapshot, from a 100 m thermal band, every 8 to 16 days of LANDSAT.
Knowledge of LST is necessary to derive the sensible heat flux exchange between the surface and the air above (H). The knowledge of H, coupled with the additional knowledge of the net radiation at the surface (Rn) and the energy flux exchange with the soil (G), make it possible to derive the remaining energy to evaporate water, i.e. the latent heat of evaporation (λE), and therefore the estimate of ET, as the residual of the energy balance (λE=Rn-H-G). Various modeling approaches are used to derive the different parameters of the energy balance, often utilizing some field measurements and inputs from other satellites. Some ET algorithms, working in conjunction with non-thermal electromagnetic bands (e.g., visible, near-infrared, etc.) from satellites often at higher spatial or temporal resolutions, deploy specific data processing (e.g., data fusion) to achieve more granular results.
The determination of ET from space is not without its challenges. Satellites are orbiting in the outer space of the atmosphere, while the determination of ET needs to occur at the land surface. Therefore, the atmosphere in between the satellites and the surface (e.g., through the presence of cloudiness, aerosols, dust, stability conditions, wind, etc.) influences the success and accuracy of ET determination. Also, the heterogeneity of the land surface (e.g., through the variability of soil-moisture content, roughness of the vegetation, topography, etc.) influences the accuracy of ET determination. Furthermore, satellites are taking instantaneous snapshots at the time of their overpass. There is a need to extend the instantaneous ET snapshot to a daily ET and, when needed, to further derive the ET of those days occurring in between satellite overpasses.
Therefore, despite the ability of SRS to better inform us about the spatial and temporal distribution of ET across the landscape, SRS carries in several uncertainties during the overall sequence of computation to determine ET. These uncertainties may tend to propagate and compound so that achieving and maintaining an acceptable degree of accuracy is not an easy task. We are facing a sort of dilemma where on the one side we cannot escape the need to use SRS if we want to cover large land-surface areas, while on the other side, and at this time, we may lack the highest degree of accuracy or consistency in accuracy required for given applications. This is an important dilemma that needs to be resolved because knowledge of the spatial distribution of ET is needed now, more than ever, as many regions face increasingly large depletion of precious water resources.
Thus, there is a need to contextualize and properly frame the way to use the SRS determination of ET. First, we need to identify the specific application and its required operational scales for which one intends to use SRS ET (e.g., water accounting at the river-basin or national scales, water allocation at the irrigation scheme scale, on-farm irrigation scheduling, etc.). For each application and scale, one must assess the commensurate spatial and temporal resolution along with the likely and acceptable uncertainty of ET (e.g., ±5%, ±10%, ±20%, etc.) in order to not obtain useless results or make meaningless or even harmful decisions. This requires that we explore what is the expected uncertainty by the various available SRS ET models and their suitability to the conditions in which the model is going to operate (e.g., cloudiness, uniformity of the land surface, daily fluctuations of weather conditions, etc.). Finally, one will try to obtain the best match between acceptable and expected uncertainties, with the understanding that the accuracy requirement for the given application may not necessarily be always satisfied. In this case, it is important to be aware of the gaps in accuracy and to adopt possible measures to mitigate and reduce such gaps. Depending on the application, we need also to be aware that sometimes even uncertain SRS results may be more useful than projections of “point estimates”.
Often, the best SRS ET results are obtained through a combination of local ground-based calibration of some model parameters (e.g., from field meteorological and/or hydrological measurements and models), including anchoring the SRS ET results to the reference evapotranspiration (ETo) obtained with field agro-meteorological station, and having highly skilled operators or reviewers of the SRS ET model.
New satellite missions with higher spatial, temporal, and spectral resolution are being launched in the coming years so that the accuracy of ET determinations is continuously improving. More advanced and dedicated meteorological measurements on the ground will also provide valuable inputs for the ET models and their testing.
Overall, the future of ET appears bright. In the meantime, it is prudent that water managers at all levels begin the adoption of recommended and dependable SRS ET models within their operations. This adoption will guide the evolution of data structures and algorithms in their accounting systems and will provide valuable feedback to the SRS ET developers.
“The postings on this site are my own and do not necessarily represent FAO’s views, positions, strategies or opinions.”